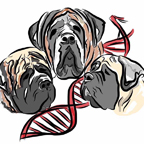
Heart Failure1
By Mark D. Kittleson, DVM, PhD, DACVIM (Cardiology), School of Veterinary Medicine, University of California, Davis
Heart failure is a clinical syndrome that occurs secondary to severe, overwhelming cardiac disease. It occurs because the heart is no longer able to maintain normal venous/capillary pressures, cardiac output, and/or systemic blood pressure. It is most commonly caused by a chronic disease that results in a severe decrease in myocardial contractility, severe regurgitation or shunting, or severe diastolic dysfunction. However, it is common to have all three abnormalities present simultaneously (but with one predominating). By far, the most common clinical manifestations seen with heart failure are directly due to edema and effusion (congestive or backward heart failure). Much less commonly, animals present because of signs referable to a decrease in cardiac output (forward heart failure). Very rarely, they present in cardiogenic shock (low blood pressure due to decreased cardiac output). This occurs because the cardiovascular system operates under a system of priorities. Its three primary functions are to maintain a normal blood pressure and normal cardiac output, both at a normal venous/capillary pressure. When the system is overwhelmed, it allows venous/capillary pressure to increase first (and so allows edema or effusion to form) and then allows cardiac output to fall. Only after cardiac output has fallen remarkably does cardiogenic shock occur. In acute heart failure, before any compensation has occurred, cardiogenic shock may predominate, but even in this situation, acute chordal rupture is the most common cause of acute heart failure in animals and results in an increased left atrial pressure and thus pulmonary edema.
Initial changes in cardiac chamber dimension (volume) or wall thickness that occur are best understood in relation to preload (the tension imposed by venous return on the ventricular walls at end-diastole) and afterload (the tension imposed on the ventricular walls at end-systole). Alterations in preload or afterload may be caused by structural cardiac abnormalities, systemic compensatory mechanisms, or both. Volume overload states, such as those that occur with chronic valvular disease/valvular insufficiencies, patent ductus arteriosus, atrial or ventricular septal defects, peripheral left-to-right shunts, anemia, or hyperthyroidism, cause an increase in preload that leads to ventricular growth and chamber enlargement (euphemistically called dilation) via eccentric myocyte hypertrophy. Pressure overload states, such as those that occur with pulmonary or systemic hypertension, and pulmonic or aortic stenosis, cause an increase in afterload (systolic intraventricular pressure) that leads to ventricular wall thickening via concentric hypertrophy. Neither volume nor pressure overload is synonymous with heart failure; either state may result in heart failure, depending on the severity of the overload and the degree of compensation.
Systolic Dysfunction
Systolic function is a broad classification of cardiac function that encompasses all of the entities in systole that are capable of altering blood flow into the aorta. It includes (but is not limited to) heart rate, myocardial contractility, preload, afterload, hypertrophy (volume and pressure overload), leaks, and shunts. Diseases that alter systolic cardiac function can become severe enough to overwhelm the ability of the cardiovascular system to compensate for the systolic dysfunction (primarily renal sodium and water retention, leading to hypervolemia, leading to increased venous return to the heart, leading to increased stretch on the myocardium, leading to myocardial growth and a larger left ventricular chamber [eccentric or volume overload hypertrophy]) and thus cause heart failure. The most common disease that alters systolic function is mitral regurgitation. Here, in systole, a portion of the blood flow that should be ejected into the aorta is ejected backward through the mitral valve from the left ventricle into the left atrium. When the regurgitation is mild (<50% of the blood flow goes backward) to moderate (50%–75% goes backward), the left ventricle is able to compensate for the leak by growing larger (volume overload hypertrophy) and increasing the total stroke volume it ejects. When regurgitation is severe (>75% backward flow), the compensatory mechanisms may become overwhelmed, resulting in an increase in left atrial pressure and so pulmonary edema. The classic example of systolic dysfunction is dilated cardiomyopathy (DCM), in which an inherent myocardial disease results in a decrease in myocardial contractility (myocardial failure). The decrease in myocardial contractility results in an increase in the end-systolic diameter/volume of the left ventricular chamber (muscle is weaker and cannot contract down as far in systole) and a decrease in myocardial contraction (the amount of wall motion [shortening fraction or fractional shortening] seen on an echocardiogram). Again, the left ventricle grows larger to compensate for this disease, but when the myocardial failure is severe, compensation can no longer maintain a normal diastolic pressure in the left ventricle (kidneys continue to retain sodium and water) and this increased pressure backs up into the left atrium, pulmonary veins, and pulmonary capillaries, creating pulmonary edema.
Diastolic Dysfunction
Diastole can be roughly divided into early myocardial relaxation and late filling that is altered primarily by compliance (1/stiffness). Most ventricular diastolic dysfunction severe enough to cause heart failure is due to myocardial fibrosis, thus due to a decrease in ventricular compliance (an increase in stiffness). When a ventricle is less compliant or stiffer than normal, for any given volume of blood that fills the chamber in diastole, the pressure is higher. This increase in diastolic pressure (when the AV valves are open) is transmitted back up into the atrium, veins, and capillary beds behind the affected ventricle, resulting in transudation of fluid and signs referable to edema or effusion. The classic example of a disease that primarily causes heart failure due to diastolic dysfunction is hypertrophic cardiomyopathy. Diastolic function is compromised to some degree by the thickening of the myocardium itself but more so by the myocardial fibrosis that builds up over time when severe disease is present. Restrictive cardiomyopathy is another classic example of diastolic dysfunction, but it is much less common. Diastolic dysfunction also occurs in pericardial diseases that cause cardiac compression (pericardial effusion, constrictive pericarditis). With pericardial disease, right heart failure (eg, ascites) predominates because systemic (eg, hepatic) capillaries leak more easily (leak profusely at a pressure of 10 mmHg) than pulmonary capillaries (which can generally withstand a pressure up to 20 mmHg without leaking).
CHF may also occur if a tumor or other anatomic obstruction impedes venous return to one or both atria. Pericardial disease or effusion leading to decreased ventricular filling may also be thought of as an extracardiac cause of CHF. Iatrogenic volume overload (ie, aggressive IV fluid therapy) can lead to edema formation in the absence of primary heart disease.
Compensatory Mechanisms
Systemic blood pressure and blood flow (and thus oxygen delivery to peripheral tissues and organs) is under strict neuroendocrine control. Compensatory mechanisms act rapidly to correct any decreases in blood flow and/or pressure. Acute compensatory mechanisms, such as increased sympathetic tone, are generally short-lived and useful only for situations that demand an acute change in cardiac function (eg, hypovolemia). Chronic mechanisms of cardiac compensation generally take over within days of the onset of a cardiac disease and are viable for years. They are responsible for the heart's ability to compensate for chronic disease. These remarkable mechanisms allow for an animal to compensate for mild, then moderate, and then even severe disease, often for years. Only at the very end of a chronic disease do they contribute to the formation of heart failure and require medical intervention.
When a decline in stroke volume occurs secondary to cardiac dysfunction, cardiac output decreases. The acute response is an increase in sympathetic tone leading to peripheral vasoconstriction, increased heart rate, and increased cardiac contractility that serve to restore cardiac output and maintain systemic blood pressure. This effect fades within days as events such as β-receptor down-regulation occur. Chronically, the renin-angiotensin-aldosterone system (RAAS) is activated. Activation is initiated by events such as decreased renal perfusion, leading to decreased sodium delivery to the macula densa (which interacts with the juxtaglomerular apparatus). The juxtaglomerular cells release renin, which converts angiotensinogen (synthesized in the liver) to angiotensin I. Angiotensin-converting enzyme (ACE) converts angiotensin I to angiotensin II, chiefly in the lungs. A separate tissue RAAS exists in the brain, vascular, and myocardial tissues, which can generate angiotensin II independently of the renal, or systemic, RAAS.
Angiotensin II has widespread effects, including stimulation of aldosterone synthesis and release from the adrenal glands, increased thirst via stimulation of antidiuretic hormone (ADH) release, increased norepinephrine and endothelin release, and stimulation of cardiac hypertrophy. Aldosterone forces the renal distal tubules to retain sodium and water. This, plus the effect of ADH, causes an increase in circulating blood volume. The increased blood volume leads to an increase in venous return to the affected ventricle. This chronic increase in preload stimulates the myocytes to add in new sarcomeres (contractile elements), leading to the growth of longer myocytes. This creates a larger ventricle (larger chamber with normal wall thickness [volume overload or eccentric hypertrophy]).
In response to these compensatory mechanisms, counter-regulatory systems are in place such as the release of atrial natriuretic peptide (ANP) from the atria, and B-type natriuretic peptide (BNP) from the atria and ventricles. ANP and BNP are released in response to stretch of the atrial and ventricular chambers. Both hormones serve to increase natriuresis (with subsequent diuresis) and decrease systemic vascular resistance, thus countering the effects of the RAAS. The effects of ANP and BNP are greatly outweighed by those of the RAAS and other systems in animals with chronic disease. Again, this is beneficial up until the end, when the RAAS continues to force sodium and water retention despite the presence of edema and effusion.
In situations when the heart must deal with higher than normal systolic intraventricular pressures (eg, subaortic stenosis, pulmonic stenosis, systemic arterial hypertension), the affected ventricle must contract against a greater force. Much like skeletal muscle when it is forced to lift a heavier weight, cardiac muscle undergoes concentric or pressure overload hypertrophy. In this situation, sarcomeres again replicate within cardiac myocytes but in parallel (side by side), to grow a wider myocyte and a thicker ventricular wall.
Cardiac Biomarkers
A biomarker is a measurable characteristic that reflects the severity or presence of some disease state. Blood pressure, cholesterol, gamma-glutamyl transferase, and BUN are all biomarkers. Studies in dogs and cats have shown that increased blood concentrations of BNP (most commonly N-terminal pro-B-type natriuretic peptide [NT-proBNP]), ANP, and endothelin-1 are indicators of cardiac disease that increase proportionately with progressive cardiac disease and CHF. Cardiac troponin I (cTnI), which is released after cardiomyocyte death, has also been evaluated as a biomarker for cardiac disease but found to be less sensitive than those mentioned above. ANP, BNP, and cTnI have also been evaluated as screening tools for occult DCM (before onset of CHF) in dogs. Increased levels of BNP were found to be highly sensitive for the detection of occult DCM, whereas ANP and cTnI were relatively less sensitive. NT-proBNP is cleaved from BNP in equal amounts in response to increased cardiac filling pressures (myocardial stretch) and ischemia, and its greater stability and longer half-life make it more suitable for use as a diagnostic biomarker. Several studies have demonstrated the usefulness of NT-proBNP in differentiating between cardiac and primary respiratory causes of dyspnea in dogs and cats. A rapid assay is available for this use in cats. Biomarkers such as NT-proBNP and troponin I should never be evaluated in isolation, because they are not 100% accurate. Instead, they should be used in concert with other diagnostic modalities.
Clinical Manifestations
The hemodynamic changes that occur in heart failure are relatively limited, as are the clinical syndromes resulting from these changes. Much depends on the location(s) of cardiac chamber failure, as well as on species differences.
Left Heart Failure:
The pulmonary veins drain into the left atrium. Left atrial pressure increases as left heart diseases worsen (eg, from regurgitant blood flow and increased circulating blood volume). An increase in left atrial pressure is transmitted to the pulmonary veins and to the pulmonary capillaries. Pulmonary capillary hydrostatic pressure continues to increase, promoting the transudation of fluid out of the capillaries and first into the lung interstitium and then into the alveoli as the pressure increase becomes more severe. Simply put, pulmonary edema develops and becomes worse as heart failure progresses. In animals, this first causes tachypnea and then dyspnea. Most owners do not notice the tachypnea and so do not seek veterinary attention until dyspnea is present. This often makes it look like the onset of the heart failure was acute, when, in fact, it was chronic.
Some dogs and a few cats and horses will cough with cardiogenic pulmonary edema. Cough is a much more common manifestation of primary lung disease in all species. Coughing is always present in dogs with chronic bronchitis. One manifestation of chronic bronchitis is airway collapse (tracheomalacia and bronchomalacia) on radiographs. Bronchomalacia appears to be evidence of chronic bronchitis in dogs. When the left atrium is enlarged, a collapsed left mainstem bronchus can often be seen above the left atrium, because the large left atrium highlights this finding. It does not appear that the large left atrium actually compresses this bronchus.
Animals with heart failure may also be exercise intolerant due to lower than normal cardiac output during exercise and/or hypoxemia, which is caused by pulmonary edema or pleural effusion. This is a rare presenting complaint in cats, because they usually do not exercise. The same is true for many dogs. In dogs, most true exercise intolerance (fatigue with marked tachypnea or dyspnea) is due to respiratory failure rather than heart failure. However, most "exercise intolerance" ends up being due to something else and is not true exercise intolerance. Instead, it is frequently an unwillingness to exercise because of other conditions, such as orthopedic disease or obesity. A dog that is truly exercise intolerant looks and sounds "out of breath." A severe decrease in cardiac output results in cold extremities (paws, ears) and can lead to total body hypothermia, especially in cats. Although syncope (transient loss of consciousness due to a transient decrease in cerebral metabolic substrate, most commonly oxygen) is not a sign of or directly due to heart failure, it may also be noted in dogs in heart failure, especially in small-breed dogs with chronic valvular disease. In many instances, the cause is unknown. However, syncope often improves once pulmonary edema is treated. In some, it is associated with coughing and is most likely a vagally mediated event (transient asystole). The syncope is frightening to the owner, but sudden death is rare unless associated with DCM or subaortic stenosis.
The diagnosis of congestive left heart failure (cardiogenic pulmonary edema) in dogs is classically made radiographically. However, the inability to take a radiograph during a deep inspiration is a diagnostic obstacle. Consequently, the caudodorsal lung fields on a lateral radiograph, where cardiogenic pulmonary edema is usually identified, often have an interstitial density that is either mistaken for pulmonary edema or hides pulmonary edema. This is exacerbated in older dogs. Most dogs with severe pulmonary edema can be identified radiographically, but those with mild to moderate edema are often problematic. In these dogs, it is often beneficial to send the dog home (if it is stable) to have the owner count the dog's sleeping respiratory rate (SRR). The owner must be taught how to count respiratory (breathing) rate and then count the rate preferably while the dog is sound asleep and in a cool environment. A normal dog has an SRR <30 breaths/min, so a rate greater than that is abnormally high (ie, the dog has tachypnea). All dogs with pulmonary edema have an increased SRR (are tachypneic). However, dogs with respiratory disease/failure can also have an increased SRR. Therefore, once an increased SRR is documented, the dog should be started on furosemide at a dosage of at least 2 mg/kg, PO, bid. If the SRR decreases, then the diagnosis of left heart failure is confirmed. The same can be done in cats for both pulmonary edema and pleural effusion. All healthy cats have an SRR <40 breaths/min, with most <30 breaths/min. Once the diagnosis is established, the owner should continue to count the SRR daily and titrate the furosemide dosage to keep the SRR within the normal range. In dogs thought to be in an impending stage of heart failure (large left atrium but no pulmonary edema), the owner can also be instructed to count the SRR daily to weekly in an attempt to identify heart failure at an early stage. Owners should also always be instructed to keep a log and bring it with them at each recheck.
In cats, left heart failure also commonly manifests as pleural effusion. The visceral pleural veins (veins on the surface of the lung) drain into the left heart rather than the right heart. This also occurs in people and dogs. The exact reason for this phenomenon is not fully understood. The pleural effusion may be a modified transudate, pseudochylous, or chylous in cats with left heart failure. A small volume of pericardial effusion can also be seen in cats with heart failure and is generally of no hemodynamic consequence (pericardiocentesis is not required). Cats also often stop eating and may stop drinking when in heart failure.
Right Heart Failure:
The right atrium receives systemic and cardiac venous and lymphatic drainage via the cranial and caudal vena cavae and the coronary sinus. Right atrial pressure increases as right heart disease worsens due to diseases such as tricuspid valve insufficiency and pulmonary hypertension. Clinical manifestations of right heart failure include jugular venous distention, hepatomegaly, pleural effusion, pericardial effusion, ascites, and peripheral edema. Dogs and cats are more likely to develop ascites (although right heart failure is uncommon in cats). Horses and cows more commonly develop subcutaneous edema ventrally. In dogs and cats, jugular venous distention may not be evident without intervention. A hepatojugular reflux test is often useful.
Management
Management of heart failure is primarily directed at controlling clinical signs related to the presence of organ edema and cavity effusion (eg, pulmonary edema, pleural or pericardial effusion, and ascites). In some animals, decreased cardiac output, and even cardiogenic shock, need to be addressed. These are accomplished through reducing preload and/or afterload (diuretics and vasodilators), improving cardiac performance (positive inotropes, positive lusitropes, antiarrhythmics), and using neurohormonal modulators (ACE inhibitors, and potentially β-blockers, aldosterone antagonists, and angiotensin II receptor blockers).
Diuretics:
The loop diuretics are the single most effective agents used to decrease circulating blood volume and reduce signs referable to edema and effusion in animals in heart failure. They act via inhibition of the Na+/K+/2 Cl– cotransporter in the thick ascending loop of Henle. This leads to increased renal sodium and chloride excretion, with subsequent free water loss. Furosemide is the most widely used loop diuretic. When administered IV, it also directly reduces pulmonary capillary wedge pressure (before onset of diuresis) via local prostaglandin synthesis, which has a vasodilatory effect. The onset of action after IV administration is 5 min, with effects peaking at 30 min and lasting ~2 hr. The onset of action after oral administration is 60 min, with peak effects seen at 1–2 hr and lasting ~6 hr.
Emergency therapy of pulmonary edema often requires high doses of IV or IM furosemide (4–8 mg/kg for dogs; 2–4 mg/kg for cats), repeated at 60- to 120-min intervals until clinical signs, usually referable to pulmonary edema (ie, tachypnea, dyspnea), are controlled. Because of the concern for dehydration, azotemia, and significant electrolyte disturbances with overzealous furosemide administration, tapering to a lower dosage as soon as the respiratory rate decreases is mandatory. Once the pulmonary edema is controlled, the dosage that keeps the sleeping respiratory rate (SRR) normal is recommended. Mild to moderate prerenal azotemia and electrolyte and acid-base disturbances (hyponatremia, hypokalemia, and a hypochloremic metabolic alkalosis) are common and are generally well tolerated, so can be safely ignored (albeit monitored carefully) as long as the animal is eating and drinking. Oral doses used to manage chronic CHF may vary significantly between animals; in theory the lowest possible dosage should be used, but care must be exercised, again via monitoring SRR, not to lower the dosage too far. In dogs, CHF is often initially controlled at dosages of 1–2 mg/kg, bid; cats are more sensitive to the development of adverse effects and generally require lower dosages (0.5–2 mg/kg every 12–24 hr). The maximal dosage in dogs is generally 4 mg/kg, PO, tid. In cats, it may be closer to 2 mg/kg, PO, tid, but dosages as high as 4 mg/kg, tid, may be required. Use of furosemide or other diuretics as sole therapy in long-term management of CHF increases RAAS activation; thus, combination with an ACE inhibitor is generally recommended.
Furosemide resistance, typically defined by persistent signs of heart failure despite dosages of 4 mg/kg, PO, tid, often develops in advanced cases of CHF. There are many causes of diuretic resistance, including remarkably severe cardiac disease, decreased delivery of the drug to the nephron (low cardiac output), activation of the RAAS (which counteracts the effects of diuresis), and hypertrophy of the distal convoluted tubular cells with consequent increases in ion transport in this region of the nephron. GI edema secondary to right CHF may decrease absorption of orally administered diuretics and contribute to diuretic resistance. Animals with resistance to chronic high doses of oral furosemide may thus have an improved diuretic response from parenteral (eg, SC) administration of the drug or from addition of other diuretic agents (“diuretic stacking”). In other cases, switching to torsemide (see below) may be the best option.
Adverse effects seen with furosemide administration are generally related to dehydration from volume depletion, reduced cardiac output and glomerular filtration rate (GFR), and electrolyte and acid-base abnormalities. Less common adverse effects include vomiting, pancreatitis, and idiosyncratic deafness with rapid IV administration. Animals with preexisting renal disease are more likely to develop adverse effects, and furosemide therapy should be reduced or temporarily withdrawn accordingly. Renal values should be monitored frequently when starting diuretic therapy (on initiation and at least 1 wk later) and should be reassessed at least every 3–6 mo during chronic administration. Some animals may remain mild to moderately azotemic (usually with a greater increase in BUN than in creatinine), which is generally tolerated provided they are eating and drinking adequately.
Torsemide is a newer loop diuretic that has better and more consistent bioavailability (better and more consistent GI absorption), has a longer duration of action (can be administered less frequently), and is more potent (requires a lower dose) than furosemide in dogs. Although there is less experience with this agent, it can be useful in management of refractory heart failure in which furosemide resistance has been documented or when furosemide is not tolerated. The starting dosage is approximately one-tenth that of the current furosemide dose (take total daily current dose of furosemide, multiply times 0.1, and divide that dose bid). Most commonly that translates into a dosage of 1–0.3 mg/kg, bid. However, dosages as high as 1 mg/kg, bid, have been used in a few dogs with ascites but only using careful upward titration.
Thiazide diuretics (eg, hydrochlorothiazide, chlorothiazide) decrease sodium resorption via inhibition of the Na+/Cl– cotransporter in the distal convoluted tubule. This results in increased sodium and water delivery to the collecting ducts and subsequent increased hydrogen and potassium excretion. Although the thiazides are relatively weak diuretics, they do exert a synergistic effect when administered with loop diuretics and can cause profound electrolyte abnormalities (particularly hypokalemia) and dehydration when used in combination if not used judiciously. Hydrochlorothiazide is more commonly used, with a recommended dosage of 1–4 mg/kg, PO, 1–2 times/day. It is also used as a combination product with the potassium-sparing diuretic spironolactone (see below), which may be dosed at 1–4 mg/kg, PO, 1–2 times/day. Many animals do not tolerate dosages at the higher end of this range, and the lowest effective dosage should be used. Chlorothiazide is dosed at 20–40 mg/kg, PO, 1–2 times/day. Thiazide diuretics are generally reserved for those cases in which resistance to furosemide has developed.
Potassium-sparing diuretics are the weakest of the diuretic class, exhibiting little to sometimes undetectable diuretic effect at standard dosages, especially when used alone in healthy dogs. Although they may produce more diuresis in a dog in heart failure, they should never be relied on to produce diuresis in an animal in heart failure. They should also never be used to replace a loop diuretic; they are used only as adjunctive (add-on) agents. Drugs in this class include the aldosterone inhibitors spironolactone and eplerenone and those that block sodium resorption at the distal tubules, triamterene and amiloride. Spironolactone is currently the only one used clinically in veterinary medicine. A large-scale clinical trial in people with heart failure in the 1990s demonstrated significant improvement in morbidity and mortality in patients with myocardial infarction administered spironolactone versus placebo, in addition to standard heart failure therapy. It was subsequently shown that the beneficial effect was all due to spironolactone's antifibrotic effects in the myocardium, not its diuretic properties. Only one discredited study from Europe has suggested benefit in dogs in heart failure. In theory, spironolactone may be of benefit in dogs with DCM, because myocardial fibrosis is a component of this disease. It is unlikely that myocardial fibrosis and diastolic dysfunction are important components of the pathophysiology of primary mitral regurgitation. In one study, spironolactone had no benefit in cats with hypertrophic cardiomyopathy before the onset of heart failure, another disease with myocardial fibrosis. Eplerenone has demonstrated a myocardial protective effect in dogs with experimentally induced myocardial infarction and heart failure.
Spironolactone is used by many veterinary cardiologists for its theoretical benefits. It may be beneficial in some dogs with refractory ascites or in those in which clinically significant hypokalemia has developed. It is dosed at 1–3 mg/kg, PO, 1–2 times/day.
Positive Inotropes:
Pimobendan is a novel inodilator (inotropic drug and vasodilator) approved by the FDA in 2007 for use in dogs with CHF related to atrioventricular valve insufficiency or DCM. It is classified as a calcium sensitizer and a phosphodiesterase (PDE) III inhibitor. The parent compound (pimobendan) is responsible for the calcium sensitization, while an active metabolite (desmethylpimobendan) is a PDE III inhibitor (~100 times more potent than pimobendan). Pimobendan sensitizes cardiomyocyte contractile proteins (mostly troponin C) to calcium, and desmethylpimobendan increases cyclic AMP and calcium cycling within the cell. Myocardial oxygen consumption is increased, as for any positive inotropic agent, in healthy dogs. Vasodilation occurs via vascular PDE III inhibition, leading to endothelial smooth muscle relaxation and calcium efflux. Vasodilation includes coronary artery vasodilation, which leads to increased coronary blood flow and increased myocardial oxygen delivery. Additional beneficial effects may include improved myocardial relaxation, anti-inflammatory and anticytokine effects, and neurohumoral modulation.
Pimobendan is not approved for use in dogs before the onset of heart failure. With regard to mitral regurgitation due to myxomatous mitral valve degeneration, a study in Beagles with mild, naturally occurring mitral regurgitation demonstrated increased valvular lesions in dogs treated with pimobendan compared with dogs treated with benazepril. Preclinical studies by the manufacturer suggest the same thing might occur in healthy dogs. A clinical study is nearing completion that looks at pimobendan vs placebo in small dogs with mitral regurgitation before onset of heart failure to determine whether it prolongs the time to onset of heart failure. A study in Doberman Pinschers with DCM but no heart failure suggested that low-dose pimobendan administration may prolong the time until the onset of heart failure or sudden death. Neither of these disparate endpoints was statistically significant on its own, but the combined endpoint was.
The combination of increased inotropy with mild afterload reduction results in improved cardiac output and reduced cardiac filling (diastolic) pressures in dogs in heart failure due to either mitral regurgitation or DCM. Clinical improvements seen with pimobendan may include improved quality of life, improved clinical scores, and a longer survival time. It is not unusual for a dog to improve clinically more than expected, and it is theorized that the drug may also have nonspecific phosphodiesterase-inhibiting properties (possibly similar to those of caffeine). Consequently, the clinical improvement seen may not always be because of improvement in heart failure. No studies to date have investigated the effects of combining pimobendan with an ACE inhibitor, but most veterinary cardiologists feel that this combination may confer additional clinical benefits. The inotropic effect of pimobendan is significantly greater than that seen with digoxin, and pimobendan has supplanted digoxin for inotropic support in CHF in dogs.
Pimobendan effectively decreases calculated pulmonary artery systolic pressure (tricuspid regurgitant jet velocity) in dogs with pulmonary hypertension secondary to mitral regurgitation. Whether this decrease is due to the expected decrease in left atrial pressure, pulmonary arterial vasodilation, or both, is unknown. There are no studies that have shown that pimobendan is beneficial in dogs with pulmonary hypertension due to causes other than left heart failure.
There is ongoing debate regarding the use of pimobendan in cats. Pimobendan is not approved for use in cats, and the package insert states that it is contraindicated in cats with hypertrophic cardiomyopathy, although several studies have suggested that the drug is safe in cats, even those with hypertrophic cardiomyopathy, when administered at the same dosage as in dogs. However, a pharmacokinetic study showed that the serum concentration of pimobendan in cats given a canine dose results in a serum pimobendan concentration 10 times that seen in dogs. Cardiac pathology is found in dogs administered 3–5 times the recommended dose. It is unlikely any species that experienced a similar calcium sensitization effect as dogs could survive a dose 10 times the recommended dose, which suggests that cats are somehow very different. This requires more investigation before rational recommendations can be made with regard to using pimobendan in cats. However, anecdotally and in one retrospective study, pimobendan has been used in cats with CHF from any cause and has been beneficial clinically. Consequently, if a cat is no longer responding to conventional heart failure drug therapy, pimobendan (1.25 mg/kg/cat, bid) may be tried.
Pimobendan is dosed at 0.2–0.3 mg/kg, PO, bid, in dogs. With progression of heart failure, many veterinary cardiologists increase the dosing frequency in dogs to tid or double the dose. Adverse effects are rare and generally seen at high dosages but may include GI upset (anorexia, diarrhea). There is no clinical evidence supporting a significant increase in arrhythmias in animals treated with pimobendan; although some studies demonstrated such a trend, others refuted this. There clearly was no increase in the incidence of sudden death in the aforementioned study of Doberman Pinschers with subclinical DCM.
The sympathomimetic amines (eg, dobutamine, dopamine) improve contractility and cardiac output via β-adrenergic agonist effects and can be valuable in the acute management of cardiogenic shock or CHF secondary to myocardial failure. Stimulation of the membrane-bound β receptor activates adenyl cyclase, leading to the production of cAMP and subsequent phosphorylation of membrane-bound calcium channels on both the sarcolemma (myocyte membrane) and sarcoplasmic reticulum. These cellular actions increase myocardial contractility and relaxation, as well as oxygen consumption. Effects on ion channels in cardiac pacemaker cells and conduction fibers lead to decreased depolarization threshold, increased heart rate, and increased conduction velocity, all of which predispose to cardiac arrhythmias. In the peripheral vasculature, mixed β1 and β2 stimulation has a negligible effect on vascular resistance, although α-adrenergic stimulation (as occurs with dopamine at higher doses) can lead to vasoconstriction.
Dobutamine is administered IV as a continuous-rate infusion at 2.5–20 mcg/kg/min diluted in 5% dextrose. Dosages >15 mcg/kg/min are rarely required and can be associated with increased tachyarrhythmias. Starting at a lower dosage with uptitration every 15–30 min as required is recommended. Concurrent ECG monitoring is strongly recommended, and if arrhythmias worsen, the dosage of dobutamine should be reduced or dobutamine should be discontinued. Because dobutamine increases conduction through the AV node, additional caution is advised in atrial fibrillation. Dobutamine may preferentially increase myocardial flow, as compared with dopamine, which tends to increase renal and mesenteric flow. Dobutamine also tends to cause less tachycardia than dopamine. Dopamine is administered as a continuous-rate infusion at 2–8 mcg/kg/min; higher dosages (>10 mcg/kg/min) are associated with vasoconstriction and tachycardia. Gradual uptitration is recommended, as with dobutamine. Both dopamine and dobutamine may cause GI upset. These agents are less commonly used in cats, although the same general treatment strategy may be followed, but starting at more conservative infusion doses (~1 mcg/kg/min) for both dobutamine and dopamine.
The bipyridine compounds (milrinone, amrinone, or inamrinone) are PDE III inhibitors. Inhibition of PDE III reduces the degradation of cAMP, with subsequent effects similar to those seen with sympathomimetic amines. These agents are generally reserved for animals with severe refractory myocardial failure, because their use is associated with a higher level of mortality than that seen with the sympathomimetic amines. Because of lack of dependence on β-receptor stimulation, PDE III inhibitors are unaffected by β-receptor downregulation or uncoupling that may occur with progressive cardiac disease and may be useful in clinical situations when benefits of sympathomimetic therapy are less than expected. Additionally, vascular PDE III inhibition and lack of α-adrenergic stimulation results in vasodilation. Adverse effects noted with PDE III inhibitors include tachycardia, tachyarrhythmias, thrombocytopenia, GI upset, and hypotension at higher dosages. Amrinone is dosed at 1–3 mg/kg, IV, or as a constant-rate infusion at 10–80 mcg/kg/min. Milrinone is dosed at 30–300 mcg/kg (loading dose), followed by 1–10 mcg/kg/min constant-rate infusion (start low and titrate).
Cardiac Glycosides:
The digitalis glycosides (eg, digoxin ) are relatively weak positive inotropes (inhibit sodium-potassium ATPase pumps on cell surface membrane) that increase vagal tone to the heart (primarily to supraventricular regions), have a narrow therapeutic range, and are associated with significantly more adverse effects than pimobendan when administered in doses that produce toxicity. Digitoxin is no longer commercially available. Although used infrequently for its inotropic effects since the introduction of pimobendan, digoxin still plays an important role in cardiac disease, particularly in atrial fibrillation or supraventricular tachycardia with concurrent CHF, because it is the only available pharmacologic agent that slows AV nodal conduction without concurrent negative inotropic effects.
Rapid (IV) digitalization commonly results in toxicity and is not recommended. Digoxin may be administered at a conservative starting dosage of 0.003–0.005 mg/kg, PO, bid. Adequate serum concentration is not achieved until the second day of administration, and a serum digoxin concentration should be checked 4–7 days after initiation of therapy, 6–8 hr after the last dose is given. The therapeutic serum concentration is in the range of 0.5–2 ng/mL. There is no added benefit to a higher serum concentration within that range, so trying to keep it in the lower end of the range is recommended. Dosage adjustments should be based on the animal’s serum digoxin concentration and clinical response. If digoxin is used in cats, it may be started at one-fourth of a 0.125-mg tablet every third day for cats <5 kg and every other day for cats >5 kg. Some larger cats may ultimately tolerate doses as high as one-fourth of a 0.125-mg tablet daily. An alcohol-based elixir form is available, although cats generally dislike the taste.
Adverse effects are increasingly likely at higher serum concentrations and generally occur in order of GI (anorexia, vomiting), cardiac (bradycardia, AV block, premature ventricular contractions), and CNS derangements. Because of its ability to slow electrical conduction as well as increase intracellular calcium, digoxin can cause almost any cardiac arrhythmia, and it is contraindicated in cases of AV block, significant bradycardia, and rapid ventricular tachycardia. If adverse effects are noted, the drug should be temporarily discontinued (usually for at least 1–2 days) and the dosage subsequently reduced by at least 50%.
Angiotensin-Converting Enzyme (ACE) Inhibitors:
ACE inhibitors competitively inhibit ACE, which converts angiotensin I to angiotensin II. This blunts the increase in systemic vascular resistance, hypertrophy, and aldosterone release caused by angiotensin II. ACE inhibitors are mild balanced vasodilators. They may reduce systemic vascular resistance up to 25%, improving cardiac output and reducing regurgitant fraction in mitral regurgitation, although these effects are rarely clinically apparent. Additional benefits include a reduction in left ventricular filling pressure and thus pulmonary edema. It is thought that the beneficial effects of ACE inhibitors are primarily due to neurohormonal modulation, in addition to hemodynamic benefits. Studies in dogs with CHF have demonstrated improved clinical scores when an ACE inhibitor was added to standard therapy (diuretics with or without digitalis glycoside), although those benefits have generally been mild and apparent only for short times. Improvements have generally been more apparent in dogs with DCM than in those with myxomatous mitral valve degeneration. A trend toward prolonged survival has been seen in some studies.
In general, veterinary cardiologists agree that an ACE inhibitor is indicated in dogs with CHF. The benefit of ACE inhibitor therapy before the onset of CHF is more controversial, although two prospective studies and one retrospective study have shown no benefit in dogs with mitral regurgitation due to myxomatous mitral valve degeneration. One retrospective study has suggested mild benefit in Doberman Pinschers with DCM. Studies of ACE inhibition in cats are limited, and none has shown a true statistical benefit of ACE inhibition beyond what is gained from standard diuretic therapy in cats with CHF. These studies had low patient numbers, however, and most cardiologists do prescribe an ACE inhibitor in addition to appropriate background therapy for cats with CHF. No benefit has been shown in delaying the progression of occult hypertrophic cardiomyopathy.
The primary serious adverse effect of ACE inhibition is related to excessive efferent glomerular arteriolar vasodilation due to an acute decrease in plasma angiotensin II concentration, resulting in a functional azotemia due to a reduced glomerular filtration rate (GFR). This is relatively rare but can happen in any animal given an ACE inhibitor. There are no known predictors in cats or dogs. Anorexia, vomiting, and lethargy (uremia) may occur, so owners should be warned to watch for these clinical signs. Renal function tests (BUN, creatinine) should be measured before starting an ACE inhibitor and again 3–7 days later. Many clinicians wait until a dog is hemodynamically stable before starting an ACE inhibitor. Although cough is a common adverse effect of ACE inhibitor therapy in people, this is not seen in dogs and cats.
Enalapril is the only approved ACE inhibitor in the USA for dogs with CHF. It is generally dosed at 0.5 mg/kg, PO, bid. ACE inhibitors are "all-or-nothing" drugs, so giving a lower or less frequent dose probably produces no effect. Long-term dosing in cats is recommended at 0.5 mg/kg/day (most commonly 2.5 mg/cat), PO. ACE inhibitors are not emergency drugs. Clinical benefits, if any, are not commonly seen before 2–3 wk. Renal values should be monitored periodically (at least every 6 mo) while on long-term ACE inhibitor therapy.
Other ACE inhibitors used for treatment of heart failure include benazepril (0.25–0.5 mg/kg/day, PO), captopril (0.5–2 mg/kg, PO, tid), and lisinopril (0.5 mg/kg, PO, 1–2 times/day). Unlike enalapril and other ACE inhibitors that are renally excreted, benazepril undergoes significant hepatobiliary elimination (as much as 50% in dogs and 85% in cats). This does not appear to reduce the incidence of functional azotemia. Whether benazepril is safer in animals with renal insufficiency is unknown.
Vasodilators:
Vasodilators exert a positive effect in CHF through dilating systemic arterioles (reduce resistance to blood flow) or systemic venodilation. The nitrates (sodium nitroprusside, nitroglycerin ointment, isosorbide dinitrate) act via the common end pathway of increased nitric oxide production, with subsequent activation of cyclic guanosine monophosphate (cGMP) and endothelial smooth muscle relaxation. Sodium nitroprusside (administered IV) is a potent mixed vasodilator, acting on both the arterial and venous systems. The combination of sodium nitroprusside with dobutamine may be especially useful in cases with cardiogenic shock and severe pulmonary edema. Although sodium nitroprusside dramatically and acutely reduces preload and afterload, its use is limited by the need for close monitoring and administration as a constant-rate infusion. The major adverse effect is systemic hypotension (with or without weakness, tachycardia, or vomiting); thus, concurrent blood pressure monitoring is recommended. However, its duration of effect is very short (1–2 min) so if problems arise, they dissipate rapidly once the infusion is stopped. Sodium nitroprusside is diluted in 5% dextrose and can be started at 1–3 mcg/kg/min, with careful uptitration every 5–10 min to desired effect, or started at 5 mcg/kg/min and the animal and/or blood pressure more carefully monitored. A dosage of 5–10 mcg/kg/min is usually sufficient to control clinical signs, and rarely is >10 mcg/kg/min required. Prolonged administration (>16 hr) increases the risk of cyanide toxicity.
If nitroprusside therapy is unavailable or undesired, nitroglycerin ointment and isosorbide dinitrate (venodilation only) might be tried, but neither is usually clinically beneficial. However, they are extremely unlikely to produce harm. Nitroglycerin is absorbed transcutaneously by the person administering the drug, so gloves must be worn during administration. It is applied at ~¼ in. per 5 kg (dogs and cats) every 6–8 hr on a hairless region such as the inner pinna or inguinal region. The latter may be preferable in animals with poor peripheral perfusion, in which the pinnae and extremities may be cool to the touch. The drug should be wiped or cleaned off after 8–12 hr or before the next dose is given. Isosorbide dinitrate is almost never used. In the one study designed to look for benefits in dogs in heart failure, none was identified when isosorbide was administered at 0.2–1 mg/kg, PO, tid. Another study showed acute benefit in dogs with mitral regurgitation when isosorbide was administered at 5 mg/kg (effect lasted 4 hr) and 10 mg/kg (effect lasted 11 hr). The largest pill size is 40 mg. Tolerance to nitrates has been demonstrated in many experimental canine models.
Hydralazine is a potent systemic arteriolar vasodilator typically reserved for dogs with mitral regurgitation due to myxomatous mitral valve degeneration that are refractory to conventional therapy, or in acute CHF when nitroprusside is unavailable. Its presumed mechanism of action is via production of vasodilatory prostaglandins. Hydralazine may reduce systemic vascular resistance up to 50%. This reduction in resistance results in the left ventricle pumping more blood flow forward through the systemic vasculature and less blood flow backward through the mitral valve into the left atrium. This decreases left atrial and pulmonary capillary pressures, reducing development of pulmonary edema. Approximately 30% of dogs vomit when receiving hydralazine; the drug generally needs to be discontinued when this happens. The recommended starting dosage in a dog in chronic heart failure is 0.5 mg/kg, PO, bid, if the animal is already on an ACE inhibitor, and 1 mg/kg, PO, bid, if not, with gradual (every 1–3 days) uptitration to effect, as high as 3 mg/kg, PO, bid. The drug is effective within 30 min, peaks in 1–3 hr, and maintains that peak for 11–13 hr. In a dog in acute, severe heart failure, a dosage of 2 mg/kg, PO, can be administered. Hydralazine is an "all-or-nothing" drug, so the primary danger is in giving too low of a dose and producing no beneficial effect. Approximately 90% of dogs respond to a dosage of 2 mg/kg. A small percentage of those dogs become weak for ~12 hr and then recover. In a dog with chronic heart failure due to mitral regurgitation, a good target would be a decrease in systolic blood pressure of 10–15 mmHg, but given the inaccuracy of blood pressure measurement in dogs this type of documentation may not be feasible. Other evidence that the dose being given is adequate is the presence of bright red mucous membranes and a decrease in murmur intensity. In cats, hydralazine can be given at a starting dose of 2.5 mg/cat, PO, bid.
Amlodipine is a calcium channel blocker with only peripheral vascular effects (no cardiac effects) and moderate to marked vasodilatory effects on systemic arterioles. Its actions are very similar to those of hydralazine (decreased mitral regurgitation and pulmonary edema) but without the adverse effects of vomiting and tachycardia. Amlodipine has a relatively slow onset of action, so its effects take 1–2 days to be noticeable. It is typically reserved for animals refractory to conventional heart failure drug therapy or for those with moderate to severe systemic hypertension. Adverse effects are generally related to hypotension and are uncommon when uptitration is done gradually. In dogs, therapy is initiated at 0.3 mg/kg/day, PO, and uptitrated every 2–3 days to a maximal dosage of 1 mg/kg/day (if needed). In cats, therapy is generally started at 0.625 mg/day (¼ of a 2.5-mg tablet) and gradually uptitrated to effect, up to as much as 1.25 mg, bid, in some cases. Because amlodipine reduces the amount of regurgitation, it may also effectively slow the progression of mitral regurgitation to heart failure in dogs. Gingival hyperplasia can occur in dogs administered amlodipine, which often necessitates discontinuation of the drug.
Phosphodiesterase type 5 (PDE-5) inhibitors (eg, sildenafil, tadalafil) are used to relax the smooth muscle in pulmonary arterioles. Their mechanism of action is somewhat similar to that seen with nitrates, with a common increase in the second messenger cGMP. PDE-5 inhibitors are used in the treatment of moderate to severe pulmonary arterial hypertension. Studies in dogs have demonstrated modest clinical improvement (reduction or cessation of syncope, improved right heart failure) with usually minimal improvement in pulmonary artery pressures, at least at lower dosages (eg, 1 mg/kg, PO, bid-tid). Anecdotally, PDE-5 inhibitors seem to provide the most notable clinical benefit to animals with syncope secondary to pulmonary hypertension. Anecdotal success has also been reported in dogs with right-to-left shunting cardiac defects in which the shunt is due to the increase in pulmonary vascular resistance. Adverse effects are uncommon but may include GI upset and hypotensive-related effects (especially when combined with nitrates, which is contraindicated). A major drawback regarding clinical use of PDE-5 inhibitors is expense, particularly in larger animals. Fake (85% in one study) sildenafil is commonly sold online. Sildenafil is administered at 1–3 mg/kg, PO, bid-tid in dogs, and at 1 mg/kg, PO, tid in cats. Tadalafil is administered at 1 mg/kg, PO, once to twice daily in dogs.
β-Adrenergic Blockers:
In people with myocardial failure (eg, DCM), β-blocker administration, with a very low starting dose titrated upward over months, results (seemingly paradoxically) in an improvement in myocardial function. To date, all attempts to replicate this phenomenon in client-owned dogs have failed. Dogs with experimentally induced heart failure have shown measurable improvements in cardiac performance after administration of metoprolol, but these were models of ischemic cardiomyopathy, which is a rare cause of DCM in dogs. Large dogs (>20 kg) with experimentally induced mitral regurgitation also have had lesser decreases in contractility over time when administered atenolol when compared with placebo.
β-Blockers are commonly administered to cats with hypertrophic cardiomyopathy, primarily to reduce systolic anterior motion of the mitral valve. However, evidence that this results in clinical improvement, biochemical improvement, or prolonged survival is lacking. Atenolol (6.25–12.5 mg/cat, PO, bid) is the β-blocker most commonly used in cats. There are limited and preliminary data associating the use of atenolol in cats with hypertrophic cardiomyopathy and CHF with a shorter survival, and consideration might be given to dosage reduction or withdrawal if CHF develops.
Nutritional Considerations:
Important metabolic changes may occur in animals with heart failure. Upregulation of the RAAS leads to increased plasma volume, largely mediated by increased sodium retention. Increased production of inflammatory cytokines such as tumor necrosis factor and interleukin 1 may promote increased metabolic demand and contribute to anorexia, thus worsening “cardiac cachexia.” Studies in people and a study in dogs have shown that patients with CHF who lost weight during the course of these studies had a poorer prognosis. In some patients, nutrient deficiencies (taurine, carnitine) have been shown to cause DCM. Decreased levels of circulating fatty acids have been documented in people and dogs with heart failure. The overall nutritional goals in the management of animals with heart failure should therefore include supplying adequate calories, modulating the production of proinflammatory cytokines, managing sodium balance, and supplementing nutrients that may be deficient.
The idea that sodium restriction reduces circulating plasma volume and preload is well established. However, sodium restriction is known to activate the RAAS, and there is continued debate as to the role of sodium restriction in animals, especially those with asymptomatic cardiac disease or mild to moderate CHF. In contrast, moderate to severe sodium restriction may be indicated in animals with severe CHF, especially in those refractory to drug therapy. It is also important to counsel owners to avoid foods and treats with high sodium content, because an acutely high sodium load (as can be seen in animals fed human snacks or table foods) may precipitate CHF in animals with compensated heart disease. For animals in mild to moderate heart failure (ISACHC Class II), moderate sodium restriction (50–80 mg/100 kcal) may be tried if the dog or cat will readily eat such a diet. In animals with severe refractory CHF, more aggressive sodium restriction (<50 mg/100 kcal) may be tried. This becomes even more of a therapeutic challenge with some animals in which cardiac cachexia is present, because lower sodium foods are often less palatable. In general, maintaining adequate caloric intake is more important than sodium restriction.
Supplementation with n-3 fatty acids has shown multiple benefits in people with CHF, and a study in dogs suggests antiarrhythmic benefits as well. These fatty acids may reduce circulating inflammatory cytokine levels and seem to improve appetite in some dogs with cardiac cachexia. Daily doses of eicosapentaenoic acid at 40 mg/kg and docosahexaenoic acid at 25 mg/kg can be tried.
Taurine supplementation is indicated in animals with documented taurine deficiency and DCM. The incidence of DCM has declined dramatically in cats since taurine deficiency was identified as a primary cause in the late 1980s. Taurine deficiency is still documented in some cats with DCM fed noncommercial diets (especially chicken, dog food, and vegetarian diets) and rarely a commercial cat food diet. Supplementation at 250 mg, PO, bid, can be started in cats while awaiting results of plasma and whole blood taurine concentrations. Dogs are able to synthesize more endogenous taurine than cats and do not have an obligatory loss in bile, so deficiency is less common in this species. However, American Cocker Spaniels with DCM uniformly have a low plasma taurine concentration and respond to taurine or taurine and carnitine supplementation. Newfoundlands are relatively predisposed to taurine deficiency, especially when fed lamb and rice or high-fiber, low-protein/taurine diets. Rarely, other breeds (mostly breeds not usually thought of as being predisposed to developing DCM) with DCM will be taurine deficient/responsive. Whole blood and plasma taurine concentrations should be obtained in any dog suspected of having a taurine-deficient cardiomyopathy, and supplementation can be started at 500–1,000 mg, PO, bid-tid, while awaiting results. Clinical improvement occurs within weeks of starting taurine supplementation in cats or dogs with DCM due to taurine deficiency. Echocardiographic improvement takes longer (2–3 mo).
L-Carnitine plays an important role in fatty acid metabolism and energy production. Carnitine deficiency has been documented in one family of Boxers and myocardial carnitine deficiency is common in dogs with DCM, but it is most likely that this deficiency is a result of cardiomyopathy, not the cause, in these dogs. Carnitine has been supplemented in other breeds with DCM but with little success. Diagnosis of carnitine deficiency is difficult and requires an endomyocardial biopsy. Supplementation is also expensive, and given our limited knowledge of the role carnitine plays in canine cardiomyopathy, supplementation is not routinely recommended. Nevertheless, supplementation can be offered at 50–100 mg/kg, PO, bid-tid, to dogs with DCM.
Coenzyme Q10 is involved with mitochondrial energy production and possesses general antioxidant properties. Anecdotal benefits of supplementation in people and dogs with DCM have been reported, but well-controlled studies are lacking, and reports are conflicting. Consequently, there is no known benefit. The dosage in dogs is 30–90 mg, PO, bid.
Oxygen Therapy:
The presence of pulmonary edema in animals with CHF increases the alveolar-arterial diffusion distance for oxygen to pulmonary capillaries and disturbs ventilation/perfusion matching. Supplemental oxygen administration increases the alveolar-arterial diffusion gradient and thus increases arterial oxygen content. Oxygen may be administered via oxygen cage, flow-by method (least preferred), nasal cannula, or oxygen collar (constructed by covering the ventral 50%–75% of an Elizabethan collar with plastic wrap and taping oxygen tubing along the ventral aspect of the collar). The oxygen cage is least stressful for the animal but is expensive, because high flows of oxygen are required to achieve therapeutic concentrations (>40% inspired oxygen). The oxygen collar has the potential to achieve a very high concentration of inspired oxygen (as much as 80%) but may require light sedation to increase patient tolerance.
Thoracocentesis:
Pleural effusion decreases the available area for alveolar ventilation and arterial oxygenation. Pleural effusion should always be excluded as the cause of dyspnea in animals in heart failure, especially cats. This is best accomplished using ultrasound. If ultrasound is unavailable, radiographs can be taken, but extreme care must be taken to avoid stressing the animal (especially cats), because stress in a dyspneic animal often results in death. If radiographs cannot be taken, a diagnostic thoracocentesis should be done. This can often be done in cats in the examination room using a butterfly catheter with the cat in sternal recumbency. If fluid is present, as much as possible should be removed. Thoracocentesis is the most effective treatment in animals with respiratory distress due to a significant volume of effusion. However, caution again should be taken in particularly stressed animals, which may require pretreatment with oxygen and light sedation. Diuretic therapy is ineffective at acutely resolving a large volume of pleural effusion.
Abdominocentesis:
Ascites may produce abdominal discomfort and worsen dyspnea by reducing available lung capacity in animals in right heart failure. Abdominocentesis should be performed at the time of initial diagnosis of right heart failure if the ascites is severe. In animals with recurrent ascites refractory to diuretic therapy, abdominocentesis may be performed every 1–4 wk to improve patient comfort and quality of life. Every attempt should be made to remove all or as much fluid as possible on each visit to prolong the time between repeat abdominocenteses. This does not result in hypoalbuminemia. Fluid can be removed manually using either a fenestrated catheter and large syringe or a suction device.
Ancillary Therapy:
Bronchodilators (theophylline, terbutaline) are generally reserved for animals with chronic airway disease, which is common in older small-breed dogs. Caution should be taken in animals with CHF, especially with tachyarrhythmias, because of the sympathomimetic effects of these agents. In dogs with cardiovascular disease and syncope, theophylline has been used with some success for its positive chronotropic effects. Dosages of theophylline and terbutaline are as previously described under treatment of bradyarrhythmias.
Cough suppressants generally should not be used if a cough is truly due to cardiogenic pulmonary edema. Instead, they are reserved for dogs with chronic bronchitis and airway collapse (malacia) and, even then, generally reserved for dogs not responsive to a corticosteroid and/or doxycycline with or without theophylline. It is common for dogs with CHF to have concomitant chronic bronchitis. Common antitussive agents used for dogs with cardiac disease include butorphanol at 0.05–0.3 mg/kg, PO, tid-qid, or hydrocodone at 0.22 mg/kg, PO, bid-tid.
Anxiolytic therapy may be used for some animals with severe respiratory distress secondary to CHF. Morphine has traditionally been recommended to alleviate anxiety in dogs with acute CHF because of its concurrent sedative and venodilating (and thus preload reducing) properties, but it also can produce respiratory depression, which can be catastrophic. The dosage of morphine in dogs is 0.1–0.25 mg/kg, SC. It may also cause nausea or vomiting. Morphine is generally avoided in cats, because it may induce agitation and dysphoria. Butorphanol is a partial opiate agonist/antagonist with minimal cardiovascular effects. A sedative dosage of 0.2–0.5 mg/kg administered IM or IV can be used in cats and dogs. Butorphanol may also be combined with a benzodiazepine (midazolam or diazepam), with the latter also dosed at 0.2–0.5 mg/kg, IM or IV. Phenothiazine tranquilizers (eg, acepromazine) may be used to alleviate severe anxiety and have the potential added benefit of producing mild systemic arteriolar dilation; however, they should be used cautiously in animals with severe hemodynamic compromise or systemic hypotension. A low dosage of 0.01–0.1 mg/kg, IM or IV, can be used.
1. Link to article ↩
Updated: 11/12/2024